金属制品的出现极大地改变和影响了人们的生活。直到今天, 各类金属材料仍在制造业,建筑业以及石油化工业等工业中扮演着重要的角色[1].但是, 金属制品产生的微生物腐蚀问题也极大地困扰着人类[2-7].
腐蚀是金属与环境之间的物理与化学作用[8].除了物理和应力造成的腐蚀外, 金属的腐蚀主要源于化学作用和电化学作用, 特别是电化学作用[9].金属的微生物腐蚀(Microbiologically influenced corrosion, MIC), 是指微生物的自身生命活动及其代谢产物直接或间接地加速金属材料腐蚀过程[10].由于涉及到生物体的参与, 它的作用机理及影响更为复杂。MIC给社会造成了巨大的经济损失, 并给人们的生产生活带来严重的危害。为了防止MIC的发生, 必须清楚地认识其机理。早期人们多采用电化学方法研究MIC, 得到的结论并不能客观完整地反映微生物腐蚀金属的真实过程。直到20世纪末期, 随着表面分析技术的发展人们对MIC的相界面过程有了更深的了解, 并逐渐意识到生物膜在MIC过程中扮演的重要作用。目前, 许多学者从生物能量学和生物电子传递方面着手, 发现微生物能利用电子,通过氧化还原中间体传递电子或者通过导电纳米线(Pili)吸收电子进行代谢活动, 从而腐蚀金属, 维持自己的生命活动。本文从生物能量学和生物电化学角度, 总结和分析微生物腐蚀金属机理研究的最新进展。
1 微生物腐蚀的生物能量学
硫酸盐还原菌(Sulfate-reducing bacteria, SRB)作为一种代表性厌氧菌, 研究其造成的金属腐蚀具有典型性, 可以得到许多具有普适性的观点和结论。这些观点和结论, 也适用于其它厌氧性细菌微生物。本文主要分析SRB对金属的腐蚀行为[9].
SRB能利用分子氢,低级脂肪醇,低级脂肪酸(如乙酸,乳酸和丙酮酸等),高级脂肪酸,脂肪烃和单芳香族化合物等作为碳源[11-16].目前人们对SRB利用硫酸盐腐蚀金属的机理已经有比较清楚的认识。图1给出了SRB细胞膜内的异化硫酸盐过程(也叫“硫酸呼吸”)。SRB能代谢硫酸盐, 主要依赖三种还原酶的作用, 即三磷酸腺苷硫酸化酶(Adenosine triphosphate sulfurylase, ATPS),腺苷硫酸盐还原酶(Adenosine phosphosulphate reductase, APR)和亚硫酸盐还原酶(Sulphite reductase, SR)[17-28].硫酸盐的还原不仅消耗ATP, 还需要电子供体向其提供电子[29-34].在碳源充足时, SRB首先利用碳源作为电子供体。如果周围环境中没有充足的碳源, SRB仍能利用其它电子供体来获得维持其生命活动的能量, 这种能量称为维持能量(Maintenance energy)。以附着在金属上的SRB生物膜为例。由于受到扩散和顶层生物膜对碳源消耗的限制, 碳源很难到达贴近金属表面的SRB生物膜, 使细菌的生长和繁殖受到有机碳源扩散的限制。在这种缺少碳源营养的条件下, SRB就不能合成其生长所必需的有机物质, 即微生物处于生长停滞阶段。但是此时SRB的能量代谢过程仍然在继续, SRB从金属获得电子来维持其生命所必需的能量。正是这一获得电子的过程, 导致金属腐蚀的发生。
顾停月和徐大可[10, 35-39]从生物能量学角度, 提出了生物阴极催化硫酸盐还原机理(Biocatalytic cathodic sulfate reduction mechanism, BCSR)。该机理认为, 当周围环境中有充足的碳源(如乳酸)时, SRB优先利用有机物质作为电子供体, 阳极发生如下的反应(1), 其中是指在25℃, pH为7, 离子浓度为1M和气体分压为105 Pa条件下, 相对于标准氢电极(Standard hydrogen electrode, SHE)的电势[12].同时SRB的生物膜分泌出细胞外多聚物(Extracellular polymeric substances, EPS)[40-42], 其主要成分是蛋白质,DNA,脂质和多糖等[43]. 由于受到扩散和顶层生物膜对碳源消耗的限制, 碳源很难到达贴近金属表面的SRB生物膜, 造成MIC受到扩散限制, 易被腐蚀的金属(例如Fe)成为唯一电子供体, 于是发生反应(2)。由反应(1)和反应(3)得到反应(4), 由反应(2)和反应(3)得到反应(5)。由公式(n为反应转移电子数; F为法拉第常数, 约等于96485 Cmol-1)得到反应(4)和反应(5)的分别为-164和-178 kJmol-1硫酸盐。由此可以看出, 反应(5)在热力学上更占优势。硫酸根在细胞膜内的活化和APS的还原反应一共需要消耗2个分子ATP(约等于消耗100 kJ能量), 所以金属Fe完全可以充当SRB的电子供体, 并且由MIC而产生的能量足够维持SRB的生命代谢活动[37, 44, 45].
(5)根据BCSR理论, 可对MIC中的许多问题有全新的认识。例如, 对于好氧细菌, 人们一般认为生物膜抑制了氧气的扩散, 从而阻碍了其与金属表面的接触, 进而起到保护金属的作用[46].但是对于厌氧细菌, 顾停月和徐大可[36, 37, 39]的BCSR理论却提出了相反的观点。他们认为, 金属表面附着的底层生物膜很难从溶液中获取碳源, 因此这些附着的生物膜必须通过腐蚀金属来获得生命体能量和代谢所需的电子, 从而加剧了金属的腐蚀。他们的实验数据表明, 当SRB生物膜处于“饥饿”状态(在没有碳源和很少量碳源的时候)导致了比碳源充足时更严重的微生物腐蚀, 说明SRB生物膜通过腐蚀金属来获得维持其生命活动的能量。这一现象从生物能量学上间接支持了BCSR理论, 而其他学者也得出了类似的结论, 证明生物能量学是研究微生物腐蚀机理的一个新的切入点[47-48].
虽然从理论上讲, 微生物可以从反应(5)中获取更多的能量, 但是在利用这些能量的过程中生物膜的导电性,表面形貌及其对金属的吸附能力等都会产生重要影响。为了更清楚地了解MIC, 必须考虑生物电化学(即细胞外电子异相传递动力学),生物膜的导电性等问题。
2 SRB造成金属腐蚀的生物电化学原理
阴极去极化理论(Cathodic depolarization theory, CDT)认为, SRB消耗阴极氢气, 使阴极去极化, 导致腐蚀电位正移, 加速了金属的腐蚀[49-53].顾停月与徐大可[54]指出, CDT理论只是BCSR理论的一种特殊情况, 只适用于那些能分泌氢化酶的SRB, 而许多不能分泌氢化酶的SRB依然能腐蚀金属。CDT理论无法解释很多现实中的MIC现象, 引起了人们对CDT理论的质疑, 并提出如下观点: (i) H2并不是MIC中胞外电子传递的唯一中介载体; (ii)只要有合适的电子传递中介体, MIC就可以发生; (iii) 在没有合适的电子传递中介体时, SRB可以直接利用电子。
Aulenta和Usher等[55, 56]通过对微生物燃料电池(Microbial fuel cell, MFC)中细胞内电子的导出机制的研究发现, 直接电子传递是微生物电子传导的重要途径。Torres等[57]指出, MFC中细胞外电子传递(Extracellular electron transfer, EET) 的主要途径有: (i)直接电子传递; (ii)基于可溶性介体的电子穿梭; (iii)细菌纳米导线。其中(i)和(iii)属于直接电子转移 (Direct electron transfer, DET), (ii)属于中介电子转移(Mediated electron transfer, MET)。徐大可和顾停月[38, 39, 58]首次将EET的概念引入到微生物腐蚀研究中, 认为微生物腐蚀的电化学机理和电子转移方式与MFC中的生物阴极(Biocathode)是相同的。他们提出的微生物腐蚀的电子转移方式, 如图2所示。
硫酸盐还原酶(Enzymes for sulfate reduction, SR); 直接电子转移(Direct electron transfer, DET); 中介电子转移(Mediated electron transfer, MET); 中介体的还原态(Reduction state of mediation, Med(red)); 中介体的氧化态(Oxidation state of mediation, Med(ox))直接电子传递机制, 主要通过希瓦氏菌(Shewanella oneidenis MR-1)和硫还原地杆菌(Geobacter metallireducens GS-15)两种导电菌进行[59, 60].大体上, 细胞存在于电子传递相关的局部位点。如已经证明的, 细胞色素c和铁硫蛋白存在于细胞外膜上, 并作为直接电子通道运输电子使电子的传递变得更加迅捷[61].Mowat和Chapman[62]鉴定出Shewanella菌具有42种细胞色素c的编码基因, 其中的14种包括4个甚至更多的亚铁血红素基因, 说明细胞色素在希瓦氏菌的胞外电子传递过程中发挥重要作用。外硫还原地杆菌装配和修饰的基因组显示, 它可能有超过100种细胞色素c[61]. 尽管细胞色素c的具体功能还不太清晰, 但已有证据表明, 多亚铁血红素在电子传递过程中起着至关重要的作用[63].
可溶性化合物的电子穿梭, 是一种间接传递方式。Lojou等[64]指出, 采用可溶性的氧化还原电子穿梭体能明显地降低其活化能, 提高电子转移速率。典型的氧化还原反应穿梭体要求水溶性好, 分子量小, 有较强的氧化还原电活性。这些中介体可直接作用于金属离子形成螯合物, 并将它们传递给细胞膜上的酶或本身充当电子载体[65]. 可溶性的氧化还原化合物包含内源性和外源性两种形式, 其中只鉴别出一小部分内源的可溶性氧化还原穿梭体, 主要有吩嗪,黄素,醌类物质,细胞色素,溶解酶,黑色素和其它介体[61] .Kaden等[66]发现, 在共培养的Geobacter sulfurreducens 和Wolinella succinogenes中半胱氨酸可以传递电子。同时, 在纯培养的G. sulfurreducens中加入半胱氨酸可加速胞外电子传递速率8-11倍[61].
Reguera等[67]在2005年首次提出纳米导线(pili)的概念, 通过导电原子力显微镜发现硫地杆菌的“菌毛蛋白”(geopili)。这种具有导电性的细丝把电子传递给金属氧化物, 将与内膜,周质或者外膜相关的蛋白电子导出到胞外空间, 通过这种渠道多血红素细胞色素能够将电子传递到菌毛上。但是, 电子流入/流出的相关机制还不是很清楚。Gorby等[68]发现, 细胞外膜色素(如十亚铁血红素细胞色素(MtrC)和细胞外膜蛋白质色素A(OmcA))被敲除后pili就失去导电能力。因此可认为, pili是由菌毛蛋白和细胞色素c的结构部分组装而成。Pili的潜在功能有: (i)作为高级细胞传递信号系统的一部分; (ii)促进胞间或中间的电子转移; (iii)与细胞的生物能量学有关[61, 69-74].上述三种EET传递方式并不是单一存在的, 它们有很好的互补与协调作用。至于哪种传递方式发挥主导作用, 要视具体微生物环境来定。
人们对胞外电子如何导入细胞的机制知之甚少, 但是可从微生物导出电子的途径中得到启示, 据推断电子导入细胞的主要方式亦是通过上述三种方式。Aulenta等[55]在2007年研究了利用甲基紫晶作为可溶性中介体, 将电子从阴极上传递给微生物。Xu等[10]在研究MIC的过程中发现, 加入黄素腺嘌呤二核苷酸(FAD)和核黄素(Riboflavin)等电子传载体, 可极大地增加了MIC导致的点蚀深度, 说明这些电子传载体大大促进了金属的腐蚀。Zhou等[75]指出, 诸如FAD这样的电子传载体, 可使那些无法和金属直接接触的微生物利用到电子。并且指出, 微生物通过与Pili共享从MIC过程中得到电子, 大大促进金属的腐蚀。据此作者提出以下观点: EET过程是微生物群落之间共生的过程, 氧化还原电子中介体和pili等EET传递机制使微生物群落中那些不具备腐蚀金属能力的细菌从那些具备腐蚀能力的微生物中获取电子并得到能量, 从而使整个微生物群落形成一个能量与信息共享的协同整体, 使微生物能更好地适应恶劣的生存环境[10, 76-79].这些工作对研究MIC的EET机理, 具有开创性作用。当然不只EET影响腐蚀电流的大小, 生物膜的导电性在对金属的腐蚀进程中也起到很重要的作用。Duan[80-82]研究了生物膜和电极之间的导电性, 发现生物膜具有良好的导电性。这说明, BCSR理论在动力学上是完全可行的。但是生物膜的形成,繁殖与脱落又是一个动态的过程, 这无疑使人们对MIC的研究工作变得更加复杂[83].
徐大可等[54]将MIC分为两类。第一类MIC是指, 贴近金属表面的底层生物膜因缺少碳源直接将金属(如Fe0)作为电子供体, 获取能量。第二类MIC是指, 微生物直接分泌一些具有腐蚀性的物质, 比如酸性物质。在生物膜下, 这些酸受到扩散的限制而造成局部H+浓度很高, 金属使严重腐蚀。Dennis等[9, 84, 85]也认为, MIC可以分为两种, 即化学微生物腐蚀(Chemical microbially influenced corrosion, CMIC) 和电化学微生物腐蚀(Electrochemical microbially influenced corrosion, EMIC)。CMIC是指SRB从周围环境中的有机物获得电子, 还原硫酸盐生成HS, 发生BCSR理论中定义的第二类微生物腐蚀, 即细菌产生的酸性分泌物腐蚀。H2S是一种强腐蚀性物质, 与金属生成腐蚀产物FeS.而FeS与其它铁的矿化物一起沉积在金属表面形成一层腐蚀产物层, 该腐蚀产物层和SRB及其代谢产物形成的生物膜一起改变金属的表面性质, 从而影响金属的腐蚀进程[86-88].EMIC更强调生物电化学过程对金属腐蚀的影响。EMIC认为, SRB直接从Fe直接获取电子造成微生物腐蚀, 这与BCSR理论中定义的第一类微生物腐蚀, 即细菌产生的传电腐蚀是一致的 [37, 85].
图3给出了Dennis提出的EMIC机理的微观图解及其涉及的相关电化学反应的电位分布状况。从图3A可以看出, 阳极Fe0腐蚀产生的电子是经细胞外膜(Outer membrane, OM),细胞周质(Periplasm, PP)和细胞质膜(Cytoplasm membrane, CM)上的蛋白质传递到SRB细胞内部。同时, SO42-被CM上的摄取硫酸盐特异蛋白(Protein for sulfate uptake, SU)所捕获, 经过硫酸盐活化酶(Enzyme for sulfate activation, SA)的活化并与其上的电子传递链偶联, 再与硫酸盐还原反应相关酶的作用下还原硫酸盐排出S2-.同时, 离子桥(Ion bridges, IB)可帮助Fe2+穿过腐蚀产物层, 然后与HCO3-和H2S作用。图3B中给出的反应电位, 都是在pH=7和标准状况下以SHE为参比得到的。
3 微生物腐蚀形成的表面膜层对腐蚀动力学的影响
许多文献曾报道了金属受到微生物腐蚀后其表面膜层的组成。Yuan[89]等研究了304不锈钢在灭菌和含有Pseudomonas两种不同介质环境下的腐蚀行为, 发现金属在受到微生物腐蚀后, 其表面膜层由一层多孔的内钝化膜和具有良好导电性的外生物膜构成。并且金属浸泡时间越长, 生物膜对内钝化膜的影响越大。Castaneda与Benetton[90]研究了SRB腐蚀不锈钢后所形成膜层的表面形态和电化学特征, 发现金属受到SRB腐蚀后表面膜层由腐蚀产物膜和含有大量EPS的生物膜构成。Bairi等[91]研究了Pseudomonas和Bacillus对D9不锈钢的腐蚀行为, 也得出生物膜下还有一层钝化膜的结论。Venzlaff等[85]分析金属在SRB影响下的电化学交流阻抗谱时, 将金属外的膜层看成一个整体。Dong等[92]用原子力显微镜(Atomic force microscopy, AFM)和扫描电子显微镜(Scanning electron microscope, SEM)观察了金属被SRB腐蚀后的表面形貌, 发现金属外覆盖着一层多相不均匀膜层。该膜层由较松散的外膜和紧密的内膜组成, 并且给出了如图4所示的生物膜结构简图。生物膜中内嵌导电的铁的硫化物, 使微孔具有良好的导电性, 从而使生物膜表现出良好的电容特性。同时, 生物膜的厚度也影响电容的大小, 使生物膜的导电性随着SRB的生长代谢而不断变化。
当金属处于微生物环境中时, 金属表面因微生物的附着而生成一层生物膜。从生态学的角度, 微生物通过生物膜腐蚀金属, 是为了更好地适应环境[93].许多学者分析了生物膜的化学成分。刘彬等[94]分析了浸泡在天然海水中14d后的不锈钢表面的生物膜成分, 发现C,O,S,Si,Mn等元素含量明显增加, 表明生物膜主要由微生物胞内物质及其有机代谢产物构成。段冶等[95]用傅里叶变换红外光谱分析仪(Fourier Transform Infrared Spectrometer, FTIR Spectrometer) 分析了Q235钢在假单胞细菌和铁细菌混合作用下的表面生物膜成分, 发现主要的吸收峰都是由聚酯糖类,脂蛋白类,细菌表面蛋白及其它细胞外聚合物官能团等引起。他们还根据能谱分析在混合体系中浸泡21 d后Q235钢的表面腐蚀产物, 能谱图上只显示出明显的Fe峰, 表明此时的腐蚀产物主要是铁的化合物。Moradi等[96]分析了Pseudoalteromonas sp.腐蚀双相不锈钢后表面生物膜的化学成分, 发现K,Cl和Na大量富集在生物膜上, 因为K,Cl和Na是构成生物膜中酶的活性元素。
生物膜的结构和形态是由周围环境因素和微生物的特性决定的[43]. Flemming等[93]认为, 生物膜是异相不均匀的, 溶液通过生物膜的多孔结构进入生物膜底部与金属直接接触。Dong等[92]研究了多电极在SRB下的腐蚀行为, 发现金属电极表面的电流分布是不均匀的, 这进一步验证了Flemming的观点[93].Xu等[97]研究了Q235钢在涂层保护下的微生物腐蚀行为, 结果也表明生物膜是异相不均匀的, 且内层的腐蚀产物层有很多裂纹。生物膜的这种异相不均匀性导致金属表面存在浓度梯度, 且其浓度梯度随着生物膜的形成,发展,成熟,死亡和脱落而变化。许多学者研究都发现, 位于生物膜下的金属与位于无菌环境中的金属相比, 更易形成点蚀和缝隙腐蚀[96, 98, 99].其原因是, 生物膜的多相异性使金属表面所处的环境各不相同, 造成金属阳极曲线的不一致, 从而发生“自催化效应”, 发生小孔腐蚀[100]. 但是Little等[101]却认为, 生物膜具有催化效应, 能增大阴极电流密度, 从而促进金属表面自钝化。Lai与Bergel[102]认为, 生物膜中的酶能催化葡萄糖转化为葡萄糖酸和H2O2.Washizu等[103]的研究结果表明, H2O2能增大阴极电流密度, 提高金属的自钝化能力。这些结果与上文提到的生物膜会加速金属点蚀的观点相矛盾。前一种理论基于生物膜物理结构及其对扩散影响方面进行考虑的, 而后一种理论考虑了生物膜对阴极的催化性能。这也进一步反映了生物膜对MIC的影响的复杂性。
4 结束语从生物能量学与生物电化学角度分析, MIC本质上是细菌适应恶劣的生存环境的一种生存策略。因此, 应该研究怎样改变微生物获取能量和电子转移的途径来缓解MIC的发生。同时认识到, 在MIC过程中微生物电子传递扮演着关键的角色。微生物的电子转移方式主要有: (1)直接电子转移; (2)通过介体转移; (3)通过pili转移。但是, 无论哪种电子转移方式都有多种酶和蛋白参与。因此可用基因工程方法抑制某些特定基因的蛋白和酶的表达, 探究金属的MIC中可能的电子传递载体, 并根据研究结果推断金属腐蚀中的电子传导机制。通过这种机制, 可寻找抑制微生物腐蚀的新靶点和新方法, 指导抗菌剂的研发,输油管道的材料选择以及抗菌材料的发展。
The authors have declared that no competing interests exist.
参考文献View Option1.U. S.Geological survey, Mineral commodity summary 2011: U. S. Geological survey, R, 2011. URLURL [本文引用:1]
2.G. H. Koch, M. P. H. Brongers, N. G. Thomposn, Y. P. Virmani, J. H. Payer, Corrosion cost and preventive strategies in the United States,R,FHWA-RD-01-156(2011)[本文引用:1]
3.J. Kruger, In Uhlig's Corrosion Hangbook, Cost of metallic corrosion, Edited by R. W. Revie(Ney Jersy, Willey, 2011) p.15[本文引用:0]
4.JIANG Bo, DU Cuiwei, The research progress of typical microbial corrosion, Petrochemical Corrosion and Protection, 25(4), 1(2008)[本文引用:0]
(蒋波, 杜翠薇, 典型微生物腐蚀的研究进展, 石油化工腐蚀与防护, 25(4), 1(2008))5.YUAN Bin, LIU Guichang, CHEN Yie, The research overview of material microbiological corrosion, Material Protection, 38(4), 38(2005)[本文引用:0]
(袁斌, 刘贵昌, 陈野, 材料微生物腐蚀的研究概况, 材料保护, 38(4), 38(2005))6.LI Yu, YU Hongwei, WEI Zheng, WANG Yuansheng, Microbiologically influenced corrosion in aviation fuel and its protection measures, Guangzhou Chemical Industry, 41(7), 27(2013)[本文引用:0]
(李瑜, 余红伟, 魏徵, 王源升, 航空燃料系统微生物危害及防护, 广州化工, 41(7), 27(2013))7.GU Caixiang, ZHU Guanjun, LI Wei, TIAN Xiaoyu, DING Shudan, The corrosion and protection measures of ship, Ship Engineering, 32(3), 1(2010)[本文引用:1]
(顾彩香, 吉贵军, 朱冠军, 李伟, 田晓禹, 丁树丹, 船舶的腐蚀与防护措施, 船舶工程, 32(3), 1(2010))8.GONG Min, YU Zuxiao, CHEN Lin, The theory of metal corrosion and corrosion control, 3rd ed., (Beijing, Chemical Industry Press, 2013) p.1[本文引用:1]
(龚敏, 余祖孝, 陈琳, 金属腐蚀理论及腐蚀控制, 第3版, (北京, 化学工业出版社, 2013)p.1)9.D. Enning, J. Garrelfs, Corrosion of iron by sufate-reducing bacteria: new views of an old problem, Applied and Environmental Microbiology, 80(4), 1226(2014)[本文引用:3]
10.P. Y. Zhang, D. K. XU, Y. C. Li, K. Yang, T. Y. Gu, Electron mediators accelerate the microbiologically influenced corrosion of 304 stainless steel by the Desulfovibrio vulgaris biofilm, Bioelectrochemistry, 101, 14(2015)[本文引用:4]
11.A. Grigoryan, G. Voordouw, Microbiology to help solve our energy needs:methanogenesis from oil and the impact on the oil-field sulfur cycle, Annals of the New York Academy Sciences, 1125, 345(2008)[本文引用:1]
12.A. A. Grigoryan, S. L. Cornish, B. Buziak, S. Lin, A. Cavallaro, J. J. Arensdorf, G. Voordouw, Competitive oxidation of volatile fatty acids by sulfate- and nitrate-reducing bacteria from an oil field in Argentia, Applied and Environmental Microbiology, 74(14), 4324(2008)[本文引用:1]
13.G. Harms, K. Zengler, R. Rabus, F. Aeckersberg, D. Minz, R. RosselloMora, F. Widdel, Anaerobic oxidation of o-xylene, m-xylene, and homologous alkylbenzenes by new types of sulfate-reducing bacteria, Applied and Environmental Microbiology, 65(3), 999(1999)[本文引用:0]
14.C. Chen, R. T. Taylor, Thermophilic biodegradation of BTEX by two consortia of anaerobic bacteria, Applied Microbiology Biotechnology, 48(1), 121(1997)[本文引用:0]
15.L. M. Gieg, I. A. Davidova, K. E. Duncan, J. M. Suflita, Methanogenesis, sulfate reduction and crude oil biodegradation in hot Alaskan oilfields, Environmental Microbiology, 12(11), 3074(2010)[本文引用:0]
16.I. A. Davidova, K. E. Duncan, O. K. Choi, J. M. Suflita, Desulfoglaeba alkanexedens gen. nov., sp. Nov., an n-alkane-degrading, sulfate-reducing bacterium, International Journal of Systematic and Evolutionary Microbiology, 56, 2737(2006)[本文引用:1]
17.F. Widdel, T. A. Hansen,In The Prokaryotes, The dissmilatory sulfate-reducing bacteria, Edited by A .Balows, H. G. Trüper, M. Dworkin, W. Harder, K. H. Schleifer(New York, Springer-Verlag, 1992) p.3379[本文引用:1]
18.F. Widdel, In Biology of Anaerobic Microorganisms. Ecological and Applied Microbiology, Microbiology and ecology of sulfate-reducing bacteria, Edited by A.J. B. Zehnder(Munich, Carl Hanser Verlag, 1988) p.469[本文引用:0]
19.R. Rabus, T. A. Hansen, F. Widdel, In The Prokaryotes, Dissimilatory sulfate-reducing and sulfur-reducing prokaryotes, Edited by S. Doworkin, E. Falkow, E. Rosenberg, K. H. Schleifer, E. Stackebrandt (Berlin,Springer, 2006) p.659[本文引用:0]
20.N. Pfennig, Metabolic diversity among the dissmilatory sulfate-reducing bacteria, Antonie Van Leeuwenhoek, 56(2), 127(1989)[本文引用:0]
21.B. Ollivier, J. L. Cayol, G. Fauque, In Sulphate-Reducing Bacteria-Environmental and Engineered Systems, Sulphate-reducing bacteria from oil field environments and deep-sea hydrothermal vents, Edited by L. L. Barton, W. Hamilton(UK, Cambridge University Press, 2007) p.305[本文引用:0]
22.J. LeGall, G. Fanque, In Biology of anaerobic Microorgansims, Dissimilatory reduction of sulfur compounds, Edited by A. J.B. Zehnder(New York, Willey, 1988) p.587[本文引用:0]
23.L. L. Keller, J. D. Wall, S. Chhabra, Methods for engineering sulfate-reducing bacteria of the genus Desulfovibrio, Methods in Enzymology, 497, 503(2011)[本文引用:0]
24.L. L. Keller, J. D. Wall, Genetics and molecular biology of the electron flow for sulfate respiration in Desulfvibrio, Frontiers in Microbiology, 2, 135(2011)[本文引用:0]
25.G. D. Fauque, J. LeGall, L. L. Barton,In Variations Autotrophic Life, Sulfur-reducing bacteria, Edited by J. M. Shively, L. L. Barton(London, Academic Press Limited, 1991) p.271[本文引用:0]
26.G. Faque, B. Ollivier, In Microbial Diversity and Bioprospecting, Anaerobes: the sulfate-reducing bacteria as an example of metabolic diversity, Edited by A. T.Bull(Washington DC, ASM Press, 2004) p.169[本文引用:0]
27.G. D. Faque, In Sulfate-Reducing Bacteria. Biotechnology Handbook, Ecology of sulfate-reducing bacteria, Edited by L. L. Barton(New York, Plenum Press, 1995) p.217[本文引用:0]
28.L. L. Barton, G. D. Faque, Biochemistry, Physiology and biotechnology of sulfate-reducing bacteria, Advances in Applied Microbiology, 68, 41(2009)[本文引用:1]
29.H. D. Jr.Peck, The ATP-dependent reduction of sulfate with hydrogen in extracts of Desulfovibrio desulfuricans, Proceedings of the National Academy Sciences, 45(5), 701(1959)[本文引用:1]
30.G. D. Fague, L. L. Barton, Hemoproteins in dissimilatory sulfate- and sulfur-reducing prokaryotes, Advances in Microbial physiology, 60, 1(2012)[本文引用:0]
31.J. Lampreia, G. Fauque, N. Speich, C. Dahl, I. Moura, H. G. Trüer, J. J. G.Moura, Spectroscopic studies on APS reductase isolated from the hyperthermophilic sulfate-reducing archaebacterium Archaeoglobus fulgidus, Biochemical and Biophysical Research Communications, 181(1), 342(1991)[本文引用:0]
32.J. Lampreia, A. S. Pereira, J. J. G.Moura, Adenylylsulfate reductases for sulfate-reducing bacteria, Methods in Enzymology, 243, 241(1994)[本文引用:0]
33.A. Lopez-Cortès, S. Bursakow, A. Figueiredo, A. E. Thapper, S. Todorovic, J. J. G.Moura, B. Ollivier, I. Moura, G. Faque, Purification and preliminary characterization of tetraheme cytochrome c3 and adenylylsulfate reductase form the peptidolytic sulfate-reducing bacterium Desulfovibrio aminophilus, Bioinorganic Chemistry Applications, 3(1-2), 81(2005)[本文引用:0]
34.G. H. Schlegel,Translated by LU Weiping,ZHOU Deqing, GUO Yanjie, MEI Baigen, General Microbiology ( Shanghai, Fudan University Press, 1990) p.305[本文引用:3]
(G. H.Schlegel着, 陆卫平, 周德庆, 郭杰炎, 梅百根译, 普通微生物学(上海, 复旦大学出版社, 1990)p.305)35.T. Y. Gu, New understandings of biocorrosion mechanisms and their classifications, Journal of Microbial & Biochemical Technology, 4, 3(2012)[本文引用:1]
36.T. Y. Gu, D. K. Xu, Carbon source starvation triggered more aggressive carbon steel by the desulfovibrio vulgaris biofilm, International Biodeterioration & Biodegradation, 91, 74(2014)[本文引用:1]
37.T. Y. Gu, D. K. Xu,“Why are some microbes corrosive and somenot?”, C,NACE-0002336(2013)[本文引用:3]
38.D. K. Xu, T. Y. Gu,“Bioenergetics explains when and why more servere MIC pitting by SRB can occur?”, C,NACE-11426(2011)[本文引用:1]
39.T. Y. Gu, D. K. Xu, “Demystifying MIC mechanisms”, C,NACE-10213(2010)[本文引用:3]
40.H. Videla, L. K. Herrera, Microbiologically influenced corrosion:.Looking to the future, International Microbiology, 8, 169(2005)[本文引用:1]
41.D. Monroe, Looking for chinks in the armor of bacterial biofilms, Plos Biology, 5(11), 2458(2007)[本文引用:0]
42.H. Castaneda, X. D. Benetton, SRB-biofilm influence in active corrosion sites formed at the steel-electrolyte interface when exposed to artificial seawater conditions, Corrosion Science, 50(4), 1169(2008)[本文引用:1]
43.QI Hanying, WANG Wenbin, ZHENG Yu, ZHU Liang, XU Xiangyang, Mechanism of biofilm formation and analysis of influencing factors, Microbiology China, 40(4), 677(2013)[本文引用:2]
(戚韩英, 汪文斌, 郑昱, 朱亮, 徐向阳, 生物膜形成机理及影响因素探究, 微生物学通报, 40(4), 677(2013))44B. K. Chastain, T. A .Kral, Zero-valent iron on Mars: An alternative energy source for methanogens, Icarus, 208(1), 198(2010)[本文引用:1]
45S. Biswas, P. Bose, Zero-valent iron-assisted autotrophic denitrification, Journal of Environmental Engineering, 131(8), 1212(2005)[本文引用:1]
46O. J. Hao, Disscusition of Modeling of anaerobic corrosion influenced by sulfate-reducing bacteria, Water Environment Research, 67(5), 875(1995)[本文引用:1]
47Y. J. Chen, Q. Tang, J. M. senko, G. Chen, B M. Z. Newby, H. Castaneda, L K. Ju, Long-term survival of Desulfovibrio vulgaris on carbon steel and pitting corrosion, Corrosion Science, 90, 89(2015)[本文引用:1]
48Y. J. Chen, R. Howdyshell, S. howdyshell, L K. Ju, Characterizing pitting corrosion caused by a long-term starving sulfate-reducing bacterium surviving on carbon steel and effects of surface roughness, Corrosion, 70(8), 767(2014)[本文引用:1]
49V. W. Kuhr, C. A. H., L. S. v. d. Vlugt, The graphitization of cast iron as an electrobiochemical process in anaerobic soil, Water, 18(16), 147(1934)[本文引用:1]
50W. P. Iverson, Direct evidence for cathodic depolarization theory of bacterial corrosion, Science, 151(3713), 986(1966)[本文引用:0]
51G. H. Booth, A. K. Tiller, Cathodic characteristics of mild steel in suspensions of sulfate-reducing bacteria, Corrosion Science, 8, 583(1968)[本文引用:0]
52J. A. Costello, Cathodic depolarization by sulphate-reducing bacteria, South African Journal of Science, 70(7), 202(1974)[本文引用:0]
53I. Pankhania, I. Moosavi, W. Hamilton, Utilization of cathodic hydrogen by Desulfvibrio vulgaris(Hidenborough), Microbiology, 132(12), 3357(1986)[本文引用:1]
54D. K. Xu, Y. C. Li, F. M. Song, T. Y. Gu, Laboratory investigation of microbiologically influenced corrosion of C1018 carbon steel by nitrate bacterium Bacillus licheniformis, Corrosion Science, 77, 385(2013)[本文引用:2]
55F. Aulenta, A. Catervi, M. Majone, S. Panero, P. Reale, S. Rossetti, Electron transfer from a solid-state electrode assisted by methyl-viologen sustains efficient microbial reductive dechlorination of TCE, Environmental Science & Technology, 41, 2554(2007)[本文引用:2]
56K. M. Usher, Critical review: Microbially influenced corrosion of buried carbon steel pipes, International Biodeterioration & Biodegradation, 93, 84(2014)[本文引用:1]
57C. I. Torres, A. K. Marcus, H Lee, P. Parameswaran, R. Krajmalnik-Brown, B. E. Rittmann, A kinetic perspective on extracellular electrons transfer by anode-respiring bacteria, FEMS Microbiology Reviews, 34(1), 3(2010)[本文引用:1]
58T. Gu, D. Xu, P. Zhang, Y. Li, A. L. Lindenberger, In Microbiology for Minerals Metals, Materials and Environment, Microbiologically influenced corrosion and its impact on metal and other materials, Edited by S. S. Abhilash, B. D. Pandey, K. A. Natarajan(Florida, CRC Press, 2015) p. 383[本文引用:3]
59C. R. Myers, K. H. Nealson, Bacterial manganese reduction and growth with managense oxide as the sole electron acceptor, Science, 240, 1319(1988)[本文引用:1]
60D. R. Lovely, E. J. P.Phillips, Novel mode of microbial energy metabolism: Organic carbon oxidation coupled to dissimilatory reduction of iron on manganese, Applied & Environmental Microbiology, 54(6), 1472(1988)[本文引用:1]
61K. Rabeay, L. Angenent, U. Schr?der, J. Keller, Translated by WANG Aijie, REN Nanqi, TAO Chunhu,Bioelectrochemical Systems: From extracellular electron transfer to biotechnological application (Beijing, Science Press, 2011) p.63[本文引用:5]
(K. Rabeay, L. Angenent, U. Schr?der, J. Keller着,王爱杰, 任南琪, 陶春虎译, 生物电化学系统: 从胞外电子传递到生物技术应用(北京, 科学出版社, 2011)p.63)62C. G. Mowat, S. K.Chapman, Multi-heme cytochromes-new structure, new chemistry, Dalton Transactions, 21, 3381(2005)[本文引用:1]
63T. Mehta, M. V. Coppi, S. E. Childers, D. R. Lovely, Outermembrane c-type cytochromes required for Fe(Ⅲ) and (Ⅳ), Oxide reduction in geobacter sulfurreducens, Applied And Enviromental Microbiology, 71(12), 8634(2005)[本文引用:1]
64E. Lojou, M. T.Giudici-Orticoni, P. Bianco, Direct electrochemistry and enzymatic activity of bacterial polyhemic cytochrome c3 incorporated in clay films, Journal of Electroanalytical Chemistry, 579, 199(2005)[本文引用:1]
65J. A. Gralnick, D. K. Newman, Extracellular respiration , Molecular Microbiology, 65(1), 1(2007)[本文引用:1]
66J. Kaden, A. S. Galushko, B. Schink, Cysteine-mediated electron transfer in syntrophic acetate oxidiation by cocultures of Geobacter sulfurreducens and Wolinella succinogenes, Archives of Microbiology, 178(1), 53(2002)[本文引用:1]
67G. Reguera, K. D. Mccarthy, T. Mehta, J. S. Nicoll, M. T. Tuominen, D. R. Lovely, Extracellular electron transfer via microbial nanowires, Nature, 435(7045), 1098(2005)[本文引用:1]
68Y. A. Gorby, S. Yanina, J. S. Mclean, K. M. Rosso, D. Moyles, A. Dohnalkova, T. J. Beveridge, I. S. Chang, B. H. Kim, K. S. Kim, D. E. Culley, S. B. Reed, M. F. Romine, D. A. Saffarini, E. A. Hill, L. Shi, D. A. Elias, D. W. kennedy, G. Pinchuk, K. Watanabe, S. I. Ishii, B. Logan, K. H. Nealson, J. K. Fredrichson, Electrically conductive bacterial nanowires produced by Shewanella oneidensis Strain MR-1 and other Microorganisms, Proceedings of The National Academy of Sciences, 103(30), 11385(2006)[本文引用:1]
69B. Erable, N. M. Duteanu, M. M. Ghangrekar, C. Dumas, K. Scott, MInireview: application of electro-active biofilms, Biofouling, 26(1), 57(2010)[本文引用:1]
70D. R. Lovely, Electrochemistry, Aunnual Review of Biochemistry, 66, 391(2012)[本文引用:0]
71N. S. Malvankar, M. Vargas, K. P. Nevin, A. E. Franks, C. Leang, B. C. Kim, K. Mester, S. F. Covalla, J. P. Johnson, V. M. Rotello, M. T. Tuominen, D. R. Lovely, Tunable metallic-like conductivity in microbial nanowire networks, Nature Nanotechnology, 6, 573(2011)[本文引用:0]
72A. E. Rotaru, P. M. Shrestha, F. Liu, M. Shrestha, D. Shrestha, M. Embree, K. Zengler, C. Wardman, K. P. Nevin, D. R. Lovely, A new model for electron flow during anaerobic digestion: direct interspecies electron transfer to methanosaeta for the reduction of carbon dioxide to methane, Energy& Environmental Science, 7(1), 408(2014)[本文引用:0]
73C. Leang, N. S. Malvankar, A. E. Franks, K. P. Nevin, D. R. Lovely, Engineering Geobacter sulfurreducens to produce a highly cohesive conductive matrix with enhanced capacity for current production, Energy & Environmental Science, 6(6), 1901(2013)[本文引用:0]
74M. Vargas, N. S. Malvankar, P. L. Tremblay, C. Leang, J. A. Smith, P. Patel, O. Synoeyenbos-West, K. P. Nevin, D. R. Lovely, Aromatic amino acids required for pili conductivity and long range extracellular electron transport in Geobacter Sulfurreducens, MBio, 4(2), 1(2013)[本文引用:1]
75M. Zhou, H. Wang, D. J. Hassett, T. Gu, Recent advances in Microbial fuel cells(MFCS) and microbial electrolysis cells (MSCS) for wastewater treatment, Bioenergy and Bioproducts, Journal of Chemical Technology and Biotechnology, 88(4), 508(2013)[本文引用:1]
76G. M. Wolfaardt, J. R. Lawrence, R. D. Robarts, S. J. Caldwell, D. E. Caldwell, Multicellular organization in a degradative biofilm community, Applied and Environment Microbiology, 60(2), 434(1994)[本文引用:1]
77G. O'Toole, H. B. Kaplan, R. kolter, Biofilm formation as microbial development, Annual Review of Microbiology, 54, 49(2000)[本文引用:0]
78E. S.McLeod, R. Macdonald, V. S. Brozel, Distribution of shewanella putrefaciens and desulfovibrio vulgaris in sulphidogenic biofilms of industrial cooling water systems determined by fluorescent in situ hybridization, Water SA, 28, 123(2004)[本文引用:0]
79G. A. James, L. Beaudette, J. W. Costerton, Interspecies bacterial intersations in biofilms, Journal of Industrial Microbiology & Biotechnology, 15(4), 257(1995)[本文引用:1]
80F. L. XU, J. Z. Duan, B. R. Hou, Electron transfer process from marine biofilms to graphite electrode in seawater, Bioelectrochemistry, 78(1), 92(2010)[本文引用:1]
81Y. Lin, J. Z. Duan, W. Zhao, Y. L. Huang, B. R. Hou, Charactersitics of hydrogen evolution and oxidation catalyzed by Desulfovibrio caledoninsis biofilm on pyrolytic graphite electrode, Electrochimica Acta, 56(26), 9041(2011)[本文引用:2]
82Y. Lin, J. Z. Duan, X. Q. Du, Y. L. Huang, B. R. Hou, Accelerated anaerobic corrosion of electroactive sulfate-reducing bacteria by electrochemical impedance spectroscopy and chronoamperometry, Electrochemistry Communications, 26, 101(2013)[本文引用:1]
83YE Qin, LI Kejuan, GUO Peipei, LIU Hongfang, Evolution of SRB biofilm and its influence on corrosion of Q235 carbon steel in oilfield sewage, Corrosion Science and Protection Technology, 25(3), 196(2013)[本文引用:1]
(叶琴, 李克娟, 郭佩佩, 刘宏芳, 油田污水中碳钢表面生物膜生长规律及腐蚀电化学行为, 腐蚀科学与防护技术, 25(3), 196-201(2013))84D. Enning, H. Venzlaff, J. Garrelfs, H. Dinh, V. Meyer, K. Mayrhofer, A. W. Hassel, M. Stratmann, Widdel F, Marine sulfate-reducing bacteria cause serious corrosion of iron under electroconductive biogenic crust, Environmental Microbiology, 14(7), 1772(2012)[本文引用:1]
85H. Venzlaff, D. Enning, J. Srinivasan, K. J. J.Mayrhofer, A. W. Hassel, F. Widdel, M. Stratmann, Accelerated Cathodic reaction in microbial corrosion of iron due to direct electron uptake by sulfate-reducing bacteria, Corrosion Science, 66, 88(2013)[本文引用:5]
86G. H. Booth, L. Elford, D. Wakerley, Corrosion of mild steel by sulphate-reducing bacteria: an alternative mechanism, British Corrosion Journal, 3(5), 242(1968)[本文引用:1]
87R. A. King, J. D. A.Miller, Corrosion by Sulphate-Reducing bacteria, Nature, 233, 491(1971)[本文引用:0]
88J. S. Smith, J. D. A.Miller, Nature of sulphides and their corrosive effect on ferrous metals: a review, British Corrosion Journal, 10(3), 136(1975)[本文引用:1]
89S. J. Yuan, S. O. Pehkonen, Y. P. Ting, E. T. Kang, K. G. Neoh, Corrosion behavior of type 304 stainless steel in a simulated sweater-based medium in the presence and absence of aerobic Psedomonas NCIMB 2021 bacteria, Industrial & Engineering Chemistry Research, 47(9), 3008(2008)[本文引用:1]
90H. Castaneda, X D. Benetton, SRB-biofilm influence in active corrosion sites formed at steel-electrolyte interface when exposed to artificial seawater conditions, Corrosion Science, 50, 1169(2008)[本文引用:1]
91L. R. Bairi, R. P. George, U. K. Mudali, Microbially induced corrosion of D9 stainless steel-zirconium metal waste form alloy under simulated geological repository, Corrosion Science, 61, 19(2012)[本文引用:1]
92Z. H. Dong, W. Shi, H. M. Ruan, Heterogeneous corrosion of mild steel under SRB-biofilm characterized by electrochemical mapping technique, Corrosion Science, 53, 2978(2011)[本文引用:2]
93H-C.Flemming, P. S. Murthy, R. Venkatesan, K.Cooksey, In Springer Series on Biofilms, Marine and industrial Biofouling, Edited by J. W. Costerton(Heidelberg, Springer-Verlag, 2009) p.1-118[本文引用:3]
94LIU Bin, DUAN Jizhou, HOU Baorong, Microbiologically influenced corrosion of 316L SS by marine biofilms in seawater, Journal of Chinese Society for Corrosion and Protection, 32(1), 49(2012)[本文引用:1]
(刘彬, 段继周, 侯保荣, 天然海水中生物膜对316L不锈钢腐蚀行为研究, 中国腐蚀与防护学报, 32(1), 49(2012))95DUAN Zhi, LI Songmei, DU Juan, LIU Jianhua, Corrosion behavior of Q235 steel in the presence of Pseudomonas and Iron Bacteria, Acta Physico-Chimica Sinica, 26(12), 3203(2010)[本文引用:1]
(段治, 李松梅, 杜鹃, 刘建华, Q235钢在假单胞菌和铁细菌混合作用下的腐蚀行为, 物理化学学报, 26(12), 3203(2010))96M. Moradi, Z. L. Son, L. J. Yang, J. J. Jiang, J. He, Effect of marine Pseudoalteromonas sp. On the microstructure and corrosion behaviour of 2205 duplex stainless steel, Corrosion Science, 84, 103(2014)[本文引用:2]
97J. Xu, K. X. Wang, C. Sun, F. H. Wang, X. M. Li, J. X. Yang, C. K. Yu, The effects of sulfate reducing bacteria on corrosion of carbon steel Q235 under simulated disbanded coating by using electrochemical impedance spectroscopy, Corrosion Science, 53, 1562(2011)[本文引用:1]
98M. Moradi, J. Duan, H. Ashassi-Sorkhabi, L. Xuan, De-alloying of 316 stainless steel in the presence of a mixture of metal-oxidizing bacteria, Corrosion Science, 53, 4282(2011)[本文引用:1]
99C. Sun, J. Xu, F. H. Wang, Interaction of sulfate-reducing bacteria and carbon steel Q235 in biofilm, Industrial & Engineering Chemistry Research, 50, 12797(2011)[本文引用:1]
100CAO Chunan, Principles of electrochemistry of corrosion,3rd ed., (Beijing, Chemical Industry Press, 2008) p.216[本文引用:1]
(曹楚南,腐蚀电化学原理, 第3版, ( 北京, 化学工业出版社, 2008)p.216)101B. J. Little, J. S. Lee, R. I. Bay, The influence of marine biofilms on Corrosion: A concise review, Electrochimica Acta, 54(1), 2-7(2008)[本文引用:1]
102M. E. Lai, A. Bergel, Direct electrochemistry of catalase on glassy carbon electrodes, Electrochemistry, 55(1-2), 157(2002)[本文引用:1]
103N. Washizu, Y. Katada, T. Kodama, Role of H2O2 in microbially influence ennoblement of open circuit potentials for type 316L stainless steel in seawater, Corrosion Science, 46, 1291(2004)[本文引用:1]
更多关于材料方面、材料腐蚀控制、材料科普等方面的国内外最新动态,我们网站会不断更新。希望大家一直关注中国腐蚀与防护网http://www.ecorr.org
责任编辑:王元
《中国腐蚀与防护网电子期刊》征订启事
投稿联系:编辑部
电话:010-62313558-806
邮箱:fsfhzy666@163.com
中国腐蚀与防护网官方 QQ群:140808414
免责声明:本网站所转载的文字、图片与视频资料版权归原创作者所有,如果涉及侵权,请第一时间联系本网删除。
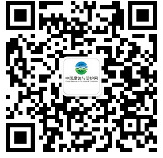
官方微信
《中国腐蚀与防护网电子期刊》征订启事
- 投稿联系:编辑部
- 电话:010-62313558-806
- 邮箱:fsfhzy666@163.com
- 中国腐蚀与防护网官方QQ群:140808414