1.4 超疏水界面的抗冰特性
对于超疏水的抗冰特性可以从3个角度思考,即去除动态水滴、控制晶核形成和降低冰的附着力。通常水滴是以动态的方式滴落到固体表面,对于高静态接触角、低动态接触角滞后的超疏水表面(Cassie-Baster状态),水滴滴落至表面时会发生反弹或者滚落,极大的减少了接触时间,避免了低温下水滴与表面接触并产生结冰。因此,动态的抗冰特性可以通过液滴在样品表面的撞击接触时间和接触过程来评价。对于具有低接触角滞后的超疏水表面,在微纳米表面结构之间存在大量的气袋。空气可以有效地充当超疏水表面和水滴之间的热屏障,令水滴与表面之间的相互作用较弱,水滴仍然保持球形,从而使液滴与表面的接触面积最小。通过这种方式,水滴很容易被外力推离表面,从而防止它们被冻结[36~38]。对于亲水表面,液滴撞击到表面会出现润湿表面的情况;对于疏水表面,液滴撞击表面的过程见图3,撞击的过程可分为4个时间点:接触时刻、最大铺展时刻、最大延伸时刻(从冠状形成开始)和脱离表面时刻[39]。在这样一个极疏水的表面液滴的最小撞击时间可达到12 ms,接触时间取决于液体的性质、表面的润湿性和水滴的运动学参数。
图3 水滴(初始直径D0 = 2 mm,冲击速度V0 =1 m·s-1)对超疏水表面的撞击过程示意图[39]
当液滴停留在固体表面,随着温度的降低,在0℃左右会很快发生结冰。如前所述,在日常环境中所见结冰现象基本是非均匀形核,在冰晶形核过程中,当突破自由能垒后晶核会稳定并很快成长成冰。超疏水材料具有抑制晶核形成的特性,主要体现为降低液滴结冰温度和延长液滴结冰时间[23]。Cassie-Baster润湿模型下的超疏水表面,其存在的微纳米分层的粗糙结构有利于捕获液滴下面的气袋。空气相比固体表面传热效率低很多,是一种很好的保温层,减缓了水滴和基体间的热交换,这种热屏障可以降低液滴的过冷程度,从而降低液滴的结冰温度。同时根据经典形核理论,接触角越大,冰核形成的自由能垒越大,较大的静态接触角也减少了液滴和表面的接触面积,减少了形核位置和概率,最终使得形核速率变慢,形核难度变大,从而延缓了液滴的结冰时间[40, 41]。Shen等[42]在金属衬底上制备了微尺度规则阵列和纳米毛层次结构,并用含氟硅烷进行修饰后获得超疏水表面,研究表明超疏水表面可以延迟结冰时间达到765 s,对晶核的形成有明显的抑制作用,见图4。微纳米结构的超疏水表面相比纳米结构的超疏水表面延迟结冰时间更长,可见微纳米结构在抗冰作用中是非常重要的。
图4 在光滑、纳米结构和微纳米结构的金属表面上的冰形成过程光学图像[42]
在低温下,对于长时间停留在表面的液滴总是会发生结冰,具有低的冰附着力的表面可以便于借助外力使冰从表面脱落,减少覆冰。冰在固体材料表面的强粘附性在很大程度上是由于极性冰分子和固体分子之间的强相互作用,这通常是由氢键、范德华力和直接静电相互作用引起的[43, 44]。研究表明[16],Cassie-Baster状态下的超疏水表面具有低的冰附着力。它会将空气储存在分层的微纳米结构中,在一定载荷下,空气提供足量的空隙,减少了冰与表面的接触面积和冰分子与固体分子之间的直接静电相互作用,达到降低粘附强度的效果。冰的粘附强度可以通过剪切或拉伸粘附试验来表征,但由于目前没有形成冰粘附试验的标准文件,研究人员均采用自行搭建的设备进行实验。
2 基于超疏水的功能性抗冰涂层的研究进展
自从发现超疏水材料具有抗冰作用后,研究人员开发了大量以超疏水为基础的抗冰涂层。然而,超疏水涂层的抗冰性能受到诸多内外因素的影响,探索解决现存问题的新方法尤为重要。近年来将不同技术、不同材料和超疏水结合在一起的新方向引起了广泛关注,如利用光热、电热等技术与超疏水结合在一起形成的多功能化抗冰涂层,这也是拓宽抗冰超疏水涂层的重要思路。基于此,本部分对这些技术和材料进行了归纳总结。
2.1 超疏水涂层
超疏水涂层具有优异的抗冰性能,正成为目前的研究热点。构成超疏水表面的条件是低表面能表面和微纳米粗糙结构,制备方法通常可分为两类:一类是典型的多步法,通常包括在低表面能表面构造微纳米粗糙度和先在表面构造粗糙结构再通过低表面能物质化学改性,具体技术有刻蚀法、沉积法、模板法、溶胶-凝胶法和层层自组装法等[17, 45~47];另一类是一步法,通过在低表面能物质成膜过程同时形成表面粗糙度,构造出微纳米结构的超疏水表面,具体技术有原位生成法、相分离法和一步喷涂法等[48~50]。如Lo等先通过化学刻蚀和水热法在Al表面制备出微纳米结构,然后将聚二甲基硅氧烷-三乙氧基硅烷(PDMS-TES)和全氟癸基三乙氧基硅烷(FD-TMS)以不同的比例混合修饰到微纳米结构表面上,PDMS-TES与铝表面为共价键结合可以提高涂层耐久性。对冰附着力和延迟结冰时间进行评估表明,即使在100次结冰/融化循环后,其对冰的粘附强度也能低至47 kPa[51]。
以各种超疏水抗冰涂层制备方法为基础,对所用材料、冰的附着力和延时结冰时间等进行总结和归纳,具体内容见表1。
表1 超疏水抗冰涂层的制备方法及各项性能表征总结
2.2 功能性光热/超疏水涂层
为扩展超疏水涂层的实际应用,近年来研究人员发展了一种新型的主动除冰和被动抗冰策略,在同时具有疏水性和光热功能的超疏水涂层方向进行了大量研究,最大限度的提高了抗冰效果。
光热材料的概念来自于太阳能集热和医学上的光热疗法,是指材料在吸收太阳光能量的同时自身可以发出足量的热量。太阳辐射的光谱范围很宽,而能量主要集中在0.25~2.5 μm之间,当太阳光束照射在光热材料表面时,一些入射光子会被其散射,而其他光子则会被吸收,被吸收的光子负责产生热[60, 61],作用过程见图5。为了确保较大的光热转换效率,需要具有大吸收效率和低发光量子产率的光热纳米材料(NP)[62]。
图5 光热材料光照生热过程原理
目前应用在光热涂层中的材料可分为金属纳米材料、碳结构材料和有机纳米材料(导电聚合物)等。不同材料由于其结构和性质不同,光热机制也是不一样的。金属纳米材料的光热机制与纳米颗粒和入射光发生表面等离子体共振作用有关,热量是由表面电流通过发热产生的弛豫产生的。碳结构材料的光热机制与π等离子共振有关(这种等离子体与碳原子之间的π键有关,属于光诱导的集体电荷运动)。导电聚合物的光热机制与光诱导载流子电流的弛豫有很大关系[62, 63]。Xie等[64]通过将PPY/ATP@hexadecylPOS悬浮液和有机硅树脂的混合物喷涂到Al板上制备出一种高效无氟、抗冰的光热超疏水涂层。研究表明,PPY/ATP@hexadecylPOS涂层在一个太阳光照下相比而言有最高的光热转换温度,10 min可升至最高温度80℃,见图6。而且在-10℃环境下对水滴延时结冰时间达330 s,在一个太阳下同样的环境延时结冰时间可达600 s,在-10℃冰的附着力只有51.6 kPa。文中的模拟室外环境研究表明该涂层有非常快的光热除冰和长效的抗冰性能[64]。
图6 ATP、PPY/ATP和PPY/ATP@十六烷基POS涂层和其在1个太阳光照下的表面温度随照射时间的变化,以及相应的ATP、PPY、PPY/ATP和PPY/ATP@十六烷基POS涂层在一定时间间隔内的红外图像[64]
基于此,对目前光热超疏水多功能涂层的制备方法、材料、冰的附着力和延时结冰时间等性能进行总结和归纳,具体内容如表2所示。
表2 光热超疏水抗冰涂层的各项性能表征总结
2.3 功能性电热/超疏水涂层
电热系统是一种广泛应用于飞机、电缆的主动防除冰策略,通过在表面制备嵌入式的电加热元件或者导电复合材料,接通电源后,会产生焦耳热量并以热传导、热对流和热辐射的方式传输热量,见图7,可达到防除冰的效果,其具有高效率、高可控性等特点,如波音和空客都应用这种技术来进行防除冰[73]。作为电热系统中的重要一环,导电复合材料得到了广泛的关注和大量的研究。近年来,具有优异电子流动性的碳材料及其衍生物成为导电复合材料的研究热点,如碳纳米管(CNTs)[74~76],高度排列的碳纳米网[77]、石墨烯[78]、石墨烯纳米带(GNP)[79]、石墨纳米片(GNR)[80]、碳纤维(CF)[81]等。如将电热复合材料和超疏水涂层结合在一起,利用超疏水涂层优异的防冰性能,辅以电热系统进行结冰后的除冰,既可以减少能量消耗,又提高了防除冰的效果。
图7 焦耳热传递示意过程
具有优异电学性能的CNTs是碳材料中应用最广泛的一种,将其与超疏水结构结合得到被动防冰和主动除冰的综合效果是研究人员的关注热点[82~85]。Chu等提出了一种重量轻、超疏水性、耐久性强、具有电热除冰性能的石墨烯基(FSGF-T200)薄膜,研究表明,FSGF-T200薄膜不但对水滴延时结冰可以延长至8倍左右,而且对冰的附着力可以降低至原先的0.2倍。此外,FSGF-T200薄膜也具有较好的电热性能,在15 V电压下可以在20 s内迅速升温到62.2℃,而且温度分布是均匀的。除霜和除冰实验发现,在15 V电压下FSGF-T200薄膜30 s内可以完全除霜,20 s内可以让冰层融化滑动,见图8[86]。
图8 FSGF-T200薄膜的电热除冰原理,在不同施加电压下的焦耳加热曲线和施加15 V直流电压后FSGF-T200的红外图像,除霜与除冰过程[86]
电热材料具有高效的除冰、解冻效率,但是往往会存在功耗大的问题,将其与超疏水技术结合在一起,可以达到一体化防冰和除冰的效果,发展高灵活度、高耐久性和低功耗的电热超疏水材料和技术也是未来抗冰涂层的一个重要研究方向。
2.4 其他复合功能性超疏水涂层
目前有较深入研究的功能性材料包括对有磁性的材料添加磁场诱导其生热,通过阳光和电压对同时具备电热光热的材料诱导其生热,作用过程见图9。在研究超疏水抗冰涂层材料的过程中,科研人员也发现将这些具有磁性的材料和同时具有光热、电热的材料等与超疏水结构结合在一起会有更好的抗冰效果,对开拓超疏水涂层的应用具有更重要的意义,也将成为未来的研究热点。
图9 涂层光生热、电生热、光电生热和磁生热作用过程
磁性纳米材料不但具有从光中吸收能量的性能,而且能够在外部交流磁场下产生自加热。可以将其光热和磁热效应的主动除冰策略与超疏水性能的被动抗冰策略相结合,制备出性能优异的抗冰涂层[72,87]。如Cheng等[72]制备出氨基功能化的磁性Fe3O4纳米颗粒,并与氟化聚合物进行交联形成功能化的涂层,研究发现目标涂层具有良好的超疏水性和润湿稳定性,超疏水表面可以将冻结时间从50 s延迟到2878 s,且对冰的粘附强度明显低于纯共聚物涂层。磁性Fe3O4的掺入对杂化薄膜带来了明显的磁场诱导加热特性,掺杂含量最多的组分在25 s可以升温至20℃以上。
将光热和电热效应的主动除冰策略与超疏水性能的被动抗冰策略相结合也是未来功能化抗冰涂层的一个创新应用方向。如Liu等采用喷涂法制备了一种由导电碳纳米管(ECNTs)和氟改性聚丙烯酸酯组成的光热@电热超疏水涂层(PESC),同时实现了防冰和除冰的效果。PESC在1.2个太阳光照下温度能达到18.6℃;当施加15 V电压时温度也可以达到42.3℃(图3.6b);对于在-30℃下有水滴的涂层表面,将阳光照明增加到0.8太阳,或电压提高到10.5 V,水滴不会出现结冰[88],见图10。
图10 PESC涂层在施加不同光强下的光照生热曲线,在施加不同电压下的焦耳生热曲线,在9.0 V电压下施加不同光强下的生热曲线,在同时施加不同电压和光强下的红外图像,在施加不同电压和光强下的水滴结冰过程和红外图[88]
3 总结与展望
自然界中常见的结冰现象,对人们的生活会产生很大影响,更重要的是对船舶、航空、电力等行业会造成严重的危害和损失。究其原理,结冰过程是在驱动力的作用下,随机自发形成不稳定的晶核,当晶核达到一个临界尺寸(超过了活化势能)就能够稳定存在并不断膨胀,最终导致整个体系的结晶。
超疏水涂层可以延缓这样的结冰过程,从而使得其在抗冰领域得到了广泛的应用。这与超疏水本身的低表面能物质和微纳米粗糙度结构有密切关系,事实上对于超疏水结构,也有Young、Wenzel和Cassie-Baster等几种不同的润湿状态,这对其性能会产生很大影响。对于超疏水的抗冰特性可以分为三个角度,即动态水滴的去除、晶核形成的控制和冰附着力的降低。然而超疏水涂层表面存在微纳米粗糙度结构容易被破坏,低表面能物质与基质的粘附力不强的缺陷,这使得其应用受到了一定程度的限制。结合已经成熟应用的电热、光热除冰技术,将此类主动除冰技术与被动抗冰的超疏水材料结合在一起,是一种非常有潜力有应用价值的抗冰策略。目前该方向研究的主要有电热超疏水涂层、光热超疏水涂层、电热+光热+超疏水涂层以及其他一些功能化的超疏水涂层。
总的来说,超疏水涂层在抗冰领域具有很大的应用价值,特别是功能化的超疏水涂层可以达到防冰和除冰的协同效果,可为极地船舶运输和科研考察等提供高效的抗冰解决方案。与此同时,制备方便、快速、低成本、对人体无毒和环境友好的超疏水抗冰涂层也是目前迫切需要解决的问题。尽管超疏水涂层将花费很长时间来实现其在工业领域的广泛应用,但这是值得我们研究和探索的。
参考文献
1 Alizadeh A, Yamada M, Li R, et al. Dynamics of ice nucleation on water repellent surfaces [J]. Langmuir, 2012, 28: 3180
doi: 10.1021/la2045256 pmid: 22235939
2 Norrstr?m A C, Bergstedt E. The impact of road de-icing salts (NaCl) on colloid dispersion and base cation pools in roadside soils [J]. Water Air Soil Poll., 2001, 127: 281
doi: 10.1023/A:1005221314856
3 Lv J Y, Song Y L, Jiang L, et al. Bio-inspired strategies for anti-icing [J]. ACS Nano, 2014, 8: 3152
doi: 10.1021/nn406522n pmid: 24592934
4 Feng L B, Yang M, Shi X T, et al. Copper-based superhydrophobic materials with long-term durability, stability, regenerability, and self-cleaning property [J]. Colloids Surf., 2016, 508A: 39
5 Wang C Z, Tang F, Li Q, et al. Spray-coated superhydrophobic surfaces with wear-resistance, drag-reduction and anti-corrosion properties [J]. Colloids Surf., 2017, 514A: 236
6 Fu S P, Sahu R P, Diaz E, et al. Dynamic study of liquid drop impact on supercooled cerium dioxide: anti-icing behavior [J]. Langmuir, 2016, 32: 6148
doi: 10.1021/acs.langmuir.6b00847
7 Pi P H, Hou K, Zhou C L, et al. Superhydrophobic Cu2S@Cu2O film on copper surface fabricated by a facile chemical bath deposition method and its application in oil-water separation [J]. Appl. Surf. Sci., 2017, 396: 566
doi: 10.1016/j.apsusc.2016.10.198
8 Zhang H F, Yin L, Li L, et al. Wettability and drag reduction of a superhydrophobic aluminum surface [J]. RSC Adv., 2016, 6: 14034
doi: 10.1039/C5RA23842K
9 Heinonen S, Huttunen-Saarivirta E, Nikkanen J P, et al. Antibacterial properties and chemical stability of superhydrophobic silver-containing surface produced by sol-gel route [J]. Colloids Surf., 2014, 453A: 149
10 Jung S, Tiwari M K, Doan N V, et al. Mechanism of supercooled droplet freezing on surfaces [J]. Nat. Commun., 2012, 3: 615
doi: 10.1038/ncomms1630 pmid: 22233625
11 Ling E J Y, Uong V, Renault-Crispo J S, et al. Reducing ice adhesion on nonsmooth metallic surfaces: wettability and topography effects [J]. ACS Appl. Mater. Interfaces, 2016, 8: 8789
doi: 10.1021/acsami.6b00187
12 Wang L, Wen M X, Zhang M Q, et al. Ice-phobic gummed tape with nano-cones on microspheres [J]. J. Mater. Chem., 2014, 2A: 3312
13 Zhang X, Shi F, Niu J, et al. Superhydrophobic surfaces: from structural control to functional application [J]. J. Mater. Chem., 2008, 18: 621
doi: 10.1039/B711226B
14 Ellinas K, Tserepi A, Gogolides E. Durable superhydrophobic and superamphiphobic polymeric surfaces and their applications: a review [J]. Adv. Colloid Interface Sci., 2017, 250: 132
15 Li S H, Huang J Y, Chen Z, et al. A review on special wettability textiles: theoretical models, fabrication technologies and multifunctional applications [J]. J. Mater. Chem., 2017, 5A: 31
16 Zhang S N, Huang J Y, Cheng Y, et al. Bioinspired surfaces with superwettability for anti-icing and ice-phobic application: concept, mechanism, and design [J]. Small, 2017, 13: 1701867
doi: 10.1002/smll.v13.48
17 Nguyen-Tri P, Tran H N, Plamondon C O, et al. Recent progress in the preparation, properties and applications of superhydrophobic nano-based coatings and surfaces: a review [J]. Prog. Org. Coat., 2019, 132: 235
doi: 10.1016/j.porgcoat.2019.03.042
18 Zeng Q H, Zhou H, Huang J X, et al. Review on the recent development of durable superhydrophobic materials for practical applications [J]. Nanoscale, 2021, 13: 11734
doi: 10.1039/d1nr01936h pmid: 34231625
19 Liu F T, Du H Z, Han Y, et al. Recent progress in the fabrication and characteristics of self-repairing superhydrophobic surfaces [J]. Adv. Mater. Interfaces, 2021, 8: 2100228
20 Webb H K, Hasan J, Truong V K, et al. Nature inspired structured surfaces for biomedical applications [J]. Curr. Med. Chem., 2011, 18: 3367
pmid: 21728964
21 Wang S T, Liu K S, Yao X, et al. Bioinspired surfaces with superwettability: new insight on theory, design, and applications [J]. Chem. Rev., 2015, 115: 8230
doi: 10.1021/cr400083y pmid: 26244444
22 Teisala H, Tuominen M, Kuusipalo J. Superhydrophobic coatings on cellulose-based materials: fabrication, properties, and applications [J]. Adv. Mater. Interfaces, 2014, 1: 1300026
doi: 10.1002/admi.v1.1
23 He H, Guo Z G. Superhydrophobic materials used for anti-icing Theory, application, and development [J]. iScience, 2021, 24: 103357
doi: 10.1016/j.isci.2021.103357
24 Li W, Zhan Y L, Yu S R. Applications of superhydrophobic coatings in anti-icing: Theory, mechanisms, impact factors, challenges and perspectives [J]. Prog. Org. Coat., 2021, 152: 106117
25 Kenzhebayeva A, Bakbolat B, Sultanov F, et al. A mini-review on recent developments in anti-icing methods [J]. Polymers, 2021, 13: 4149
doi: 10.3390/polym13234149
26 Gohari B, Russell K, Hejazi V, et al. Role of water solidification concepts in designing nano-textured anti-icing surfaces [J]. J. Phys. Chem., 2017, 121B: 7527
27 Zhang Z S, Liu X Y. Control of ice nucleation: freezing and antifreeze strategies [J]. Chem. Soc. Rev., 2018, 47: 7116
doi: 10.1039/c8cs00626a pmid: 30137078
28 Moore E B, Molinero V. Structural transformation in supercooled water controls the crystallization rate of ice [J]. Nature, 2011, 479: 506
doi: 10.1038/nature10586
29 Li Q, Guo Z G. Fundamentals of icing and common strategies for designing biomimetic anti-icing surfaces [J]. J. Mater. Chem., 2018, 6A: 13549
30 Lin N B, Liu X Y. Correlation between hierarchical structure of crystal networks and macroscopic performance of mesoscopic soft materials and engineering principles [J]. Chem. Soc. Rev., 2015, 44: 7881
doi: 10.1039/c5cs00074b pmid: 26214062
31 Liu X Y. Interfacial effect of molecules on nucleation kinetics [J]. J. Phys. Chem., 2001, 105B: 11550
32 Liu X Y, Maiwa K, Tsukamoto K. Heterogeneous two-dimensional nucleation and growth kinetics [J]. J. Chem. Phys., 1997, 106: 1870
33 Fletcher N H. Size effect in heterogeneous nucleation [J]. J. Chem. Phys., 1958, 29: 572
34 Liu X Y. A new kinetic model for three-dimensional heterogeneous nucleation [J]. J. Chem. Phys., 1999, 111: 1628
doi: 10.1063/1.479391
35 Schutzius T M, Jung S, Maitra T, et al. Physics of icing and rational design of surfaces with extraordinary icephobicity [J]. Langmuir, 2015, 31: 4807
doi: 10.1021/la502586a pmid: 25346213
36 Vandadi A, Zhao L, Cheng J T. Resistant energy analysis of self-pulling process during dropwise condensation on superhydrophobic surfaces [J]. Nanoscale Adv., 2019, 1: 1136
doi: 10.1039/c8na00237a pmid: 36133189
37 Liu B, Zhang K Q, Tao C, et al. Strategies for anti-icing: low surface energy or liquid-infused? [J]. RSC Adv., 2016, 6: 70251
doi: 10.1039/C6RA11383D
38 Barthwal S, Lim S H. Rapid fabrication of a dual-scale micro-nanostructured superhydrophobic aluminum surface with delayed condensation and ice formation properties [J]. Soft Matter, 2019, 15: 7945
doi: 10.1039/c9sm01256g pmid: 31544192
39 Shen Y, Tao J, Tao H, et al. Approaching the theoretical contact time of a bouncing droplet on the rational macrostructured superhydrophobic surfaces [J]. Appl. Phys. Lett., 2015, 107: 111604
40 Liu W P, Wang C M, Zhang L J, et al. Exfoliation of amorphous phthalocyanine conjugated polymers into ultrathin nanosheets for highly efficient oxygen reduction [J]. J. Mater. Chem., 2019, 7A: 3112
41 Yin Z Z, Xue M S, Luo Y D, et al. Excellent static and dynamic anti-icing properties of hierarchical structured ZnO superhydrophobic surface on Cu substrates [J]. Chem. Phys. Lett., 2020, 755: 137806
doi: 10.1016/j.cplett.2020.137806
42 Shen Y Z, Tao J, Tao H J, et al. Superhydrophobic Ti6Al4V surfaces with regular array patterns for anti-icing applications [J]. RSC Adv., 2015, 5: 32813
doi: 10.1039/C5RA01365H
43 Jia Z F, Shen Y Z, Tao J, et al. Understanding the solid-ice interface mechanism on the hydrophobic nano-pillar structure epoxy surface for reducing ice adhesion [J]. Coatings, 2020, 10: 1043
doi: 10.3390/coatings10111043
44 Zhang Y F, Zhang L Q, Xiao Z, et al. Fabrication of robust and repairable superhydrophobic coatings by an immersion method [J]. Chem. Eng. J., 2019, 369: 1
doi: 10.1016/j.cej.2019.03.021
45 Shen Y Z, Wang G Y, Zhu C L, et al. Petal shaped nanostructures planted on array micro-patterns for superhydrophobicity and anti-icing applications [J]. Surf. Coat. Technol., 2017, 319: 286
46 Jin H Y, Nie S C, Li Y F, et al. Investigation of the static icing property for super-hydrophobic coatings on aluminium [J]. Mater. Tehnol., 2017, 51: 789
doi: 10.17222/mit
47 Qian C L, Li Q, Chen X M. Droplet impact on the cold elastic superhydrophobic membrane with low ice adhesion [J]. Coatings, 2020, 10: 964
doi: 10.3390/coatings10100964
48 Luo Z Z, Zhang Z Z, Wang W J, et al. Various curing conditions for controlling PTFE micro/nano-fiber texture of a bionic superhydrophobic coating surface [J]. Mater. Chem. Phys., 2010, 119: 40
doi: 10.1016/j.matchemphys.2009.07.039
49 Luo Z Z, Zhang Z Z, Hu L T, et al. Stable bionic superhydrophobic coating surface fabricated by a conventional curing process [J]. Adv. Mater., 2008, 20: 970
doi: 10.1002/adma.v20:5
50 Lin Y B, Chen H F, Wang G Y, et al. Recent progress in preparation and anti-icing applications of superhydrophobic coatings [J]. Coatings, 2018, 8: 208
doi: 10.3390/coatings8060208
51 Lo T N H, Lee J, Hwang H S, et al. Nanoscale coatings derived from fluoroalkyl and PDMS alkoxysilanes on rough aluminum surfaces for improved durability and anti-icing properties [J]. ACS Appl. Nano Mater., 2021, 4: 7493
doi: 10.1021/acsanm.1c01526
52 Shen Y Z, Wu Y, Tao J, et al. Spraying fabrication of durable and transparent coatings for anti-icing application: dynamic water repellency, icing delay, and ice adhesion [J]. ACS Appl. Mater. Interfaces, 2019, 11: 3590
doi: 10.1021/acsami.8b19225
53 Pan L, Wang F, Pang X F, et al. Superhydrophobicity and anti-icing of CF/PEEK composite surface with hierarchy structure [J]. J. Mater. Sci., 2019, 54: 14728
doi: 10.1007/s10853-019-03956-0
54 Xie H, Zhao X, Li B C, et al. Waterborne, non-fluorinated and durable anti-icing superhydrophobic coatings based on diatomaceous earth [J]. New J. Chem., 2021, 45: 10409
doi: 10.1039/D1NJ01307F
55 Li K Q, Zeng X R, Li H Q, et al. A study on the fabrication of superhydrophobic iron surfaces by chemical etching and galvanic replacement methods and their anti-icing properties [J]. Appl. Surf. Sci., 2015, 346: 458
56 Wu Y L, She W, Shi D A, et al. An extremely chemical and mechanically durable siloxane bearing copolymer coating with self-crosslinkable and anti-icing properties [J]. Composites, 2020, 195B: 108031
57 Qi Y L, Yang Z B, Chen T T, et al. Fabrication of superhydrophobic surface with desirable anti-icing performance based on micro/nano-structures and organosilane groups [J]. Appl. Surf. Sci., 2020, 501: 144165
doi: 10.1016/j.apsusc.2019.144165
58 Tan X Y, Huang Z T, Jiang L H, et al. A simple fabrication of superhydrophobic PVDF/SiO2 coatings and their anti-icing properties [J]. J. Mater. Res., 2021, 36: 637
59 Tong W, Xiong D S, Wang N, et al. Mechanically robust superhydrophobic coating for aeronautical composite against ice accretion and ice adhesion [J]. Composites, 2019, 176B: 107267
60 Ding Z, Qi C, Wang Y X, et al. Spectrally selective absorption coatings and their applications: A review [J]. Sustain. Energy Technol. Assess., 2022, 52: 102031
61 Xu K, Du M, Hao L, et al. A review of high-temperature selective absorbing coatings for solar thermal applications [J]. J. Materiomics, 2020, 6: 167
doi: 10.1016/j.jmat.2019.12.012
62 Jaque D, Maestro L M, del Rosal B, et al. Nanoparticles for photothermal therapies [J]. Nanoscale, 2014, 6: 9494
doi: 10.1039/c4nr00708e pmid: 25030381
63 Liu Y B, Wu Y, Liu S J, et al. Material strategies for ice accretion prevention and easy removal [J]. ACS Mater. Lett., 2022, 4: 246
64 Xie H, Wei J F, Duan S Y, et al. Non-fluorinated and durable photothermal superhydrophobic coatings based on attapulgite nanorods for efficient anti-icing and deicing [J]. Chem. Eng. J., 2022, 428: 132585
65 Zhao W R, Xiao L, He X Y, et al. Moth-eye-inspired texturing surfaces enabled self-cleaning aluminum to achieve photothermal anti-icing [J]. Opt. Laser Technol., 2021, 141: 107115
doi: 10.1016/j.optlastec.2021.107115
66 Ma L W, Wang J K, Zhao F T, et al. Plasmon-mediated photothermal and superhydrophobic TiN-PTFE film for anti-icing/deicing applications [J]. Compos. Sci. Technol., 2019, 181: 107696
doi: 10.1016/j.compscitech.2019.107696
67 Xie H, Xu W H, Fang C, et al. Efficient and economical approach for flexible photothermal icephobic copper mesh with robust superhydrophobicity and active deicing property [J]. Soft Matter, 2021, 17: 1901
doi: 10.1039/d0sm01930e pmid: 33416069
68 Xue C H, Li H G, Guo X J, et al. Superhydrophobic anti-icing coatings with self-deicing property using melanin nanoparticles from cuttlefish juice [J]. Chem. Eng. J., 2021, 424: 130553
doi: 10.1016/j.cej.2021.130553
69 Liu Y B, Wu Y, Liu Y Z, et al. Robust photothermal coating strategy for efficient ice removal [J]. ACS Appl. Mater. Interfaces, 2020, 12: 46981
doi: 10.1021/acsami.0c13367
70 Hu J H, Jiang G. Superhydrophobic coatings on iodine doped substrate with photothermal deicing and passive anti-icing properties [J]. Surf. Coat. Technol., 2020, 402: 126342
doi: 10.1016/j.surfcoat.2020.126342
71 Jiang G, Chen L, Zhang S D, et al. Superhydrophobic SiC/CNTs coatings with photothermal deicing and passive anti-icing properties [J]. ACS Appl. Mater. Interfaces, 2018, 10: 36505
doi: 10.1021/acsami.8b11201
72 Cheng T T, He R, Zhang Q H, et al. Magnetic particle-based super-hydrophobic coatings with excellent anti-icing and thermoresponsive deicing performance [J]. J. Mater. Chem., 2015, 3A: 21637
73 Parent O, Ilinca A. Anti-icing and de-icing techniques for wind turbines: Critical review [J]. Cold Reg. Sci. Technol., 2011, 65: 88
doi: 10.1016/j.coldregions.2010.01.005
74 Jung D, Kim D, Lee K H, et al. Transparent film heaters using multi-walled carbon nanotube sheets [J]. Sensors Actuat., 2013, 199A: 176
75 Im H, Jang E Y, Choi A, et al. Enhancement of heating performance of carbon nanotube sheet with granular metal [J]. ACS Appl. Mater. Interfaces, 2012, 4: 2338
doi: 10.1021/am300477u
76 Chu H T, Zhang Z C, Liu Y J, et al. Self-heating fiber reinforced polymer composite using meso/macropore carbon nanotube paper and its application in deicing [J]. Carbon, 2014, 66: 154
doi: 10.1016/j.carbon.2013.08.053
77 Yao X D, Hawkins S C, Falzon B G. An advanced anti-icing/de-icing system utilizing highly aligned carbon nanotube webs [J]. Carbon, 2018, 136: 130
doi: 10.1016/j.carbon.2018.04.039
78 Vertuccio L, De Santis F, Pantani R, et al. Effective de-icing skin using graphene-based flexible heater [J]. Composites, 2019, 162B: 600
79 Redondo O, Prolongo S G, Campo M, et al. Anti-icing and de-icing coatings based Joule's heating of graphene nanoplatelets [J]. Compos. Sci. Technol., 2018, 164: 65
doi: 10.1016/j.compscitech.2018.05.031
80 Raji A R O, Varadhachary T, Nan K W, et al. Composites of graphene nanoribbon stacks and epoxy for joule heating and deicing of surfaces [J]. ACS Appl. Mater. Interfaces, 2016, 8: 3551
doi: 10.1021/acsami.5b11131
81 Kim T, Chung D D L. Carbon fiber mats as resistive heating elements [J]. Carbon, 2003, 41: 2436
doi: 10.1016/S0008-6223(03)00288-4
82 Zhao Z H, Chen H W, Liu X L, et al. Novel sandwich structural electric heating coating for anti-icing/de-icing on complex surfaces [J]. Surf. Coat. Technol., 2020, 404: 126489
doi: 10.1016/j.surfcoat.2020.126489
83 Zhao Z H, Chen H W, Liu X L, et al. Development of high-efficient synthetic electric heating coating for anti-icing/de-icing [J]. Surf. Coat. Technol., 2018, 349: 340
84 Peng M, Liao Z J, Qi J, et al. Nonaligned carbon nanotubes partially embedded in polymer matrixes: a novel route to superhydrophobic conductive surfaces [J]. Langmuir, 2010, 26: 13572
doi: 10.1021/la101827c pmid: 20695606
85 Wang F X, Tay T E, Sun Y Y, et al. Low-voltage and -surface energy SWCNT/poly(dimethylsiloxane) (PDMS) nanocomposite film: Surface wettability for passive anti-icing and surface-skin heating for active deicing [J]. Compos. Sci. Technol., 2019, 184: 107872
doi: 10.1016/j.compscitech.2019.107872
86 Chu Z M, Jiao W C, Huang Y F, et al. FDTS-modified SiO2/rGO wrinkled films with a micro-nanoscale hierarchical structure and anti-icing/deicing properties under condensation condition [J]. Adv. Mater. Interfaces, 2020, 7: 1901446
doi: 10.1002/admi.v7.1
87 Zhu R F, Liu M M, Hou Y Y, et al. One-pot preparation of fluorine-free magnetic superhydrophobic particles for controllable liquid marbles and robust multifunctional coatings [J]. ACS Appl. Mater. Interfaces, 2020, 12: 17004
doi: 10.1021/acsami.9b22268
88 Liu Y B, Xu R N, Luo N, et al. All-day anti-icing/De-icing coating by solar-thermal and electric-thermal effects [J]. Adv. Mater. Technol., 2021, 6: 2100371
doi: 10.1002/admt.v6.11
免责声明:本网站所转载的文字、图片与视频资料版权归原创作者所有,如果涉及侵权,请第一时间联系本网删除。
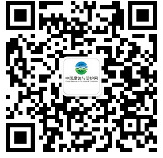
官方微信
《腐蚀与防护网电子期刊》征订启事
- 投稿联系:编辑部
- 电话:010-62316606
- 邮箱:fsfhzy666@163.com
- 腐蚀与防护网官方QQ群:140808414